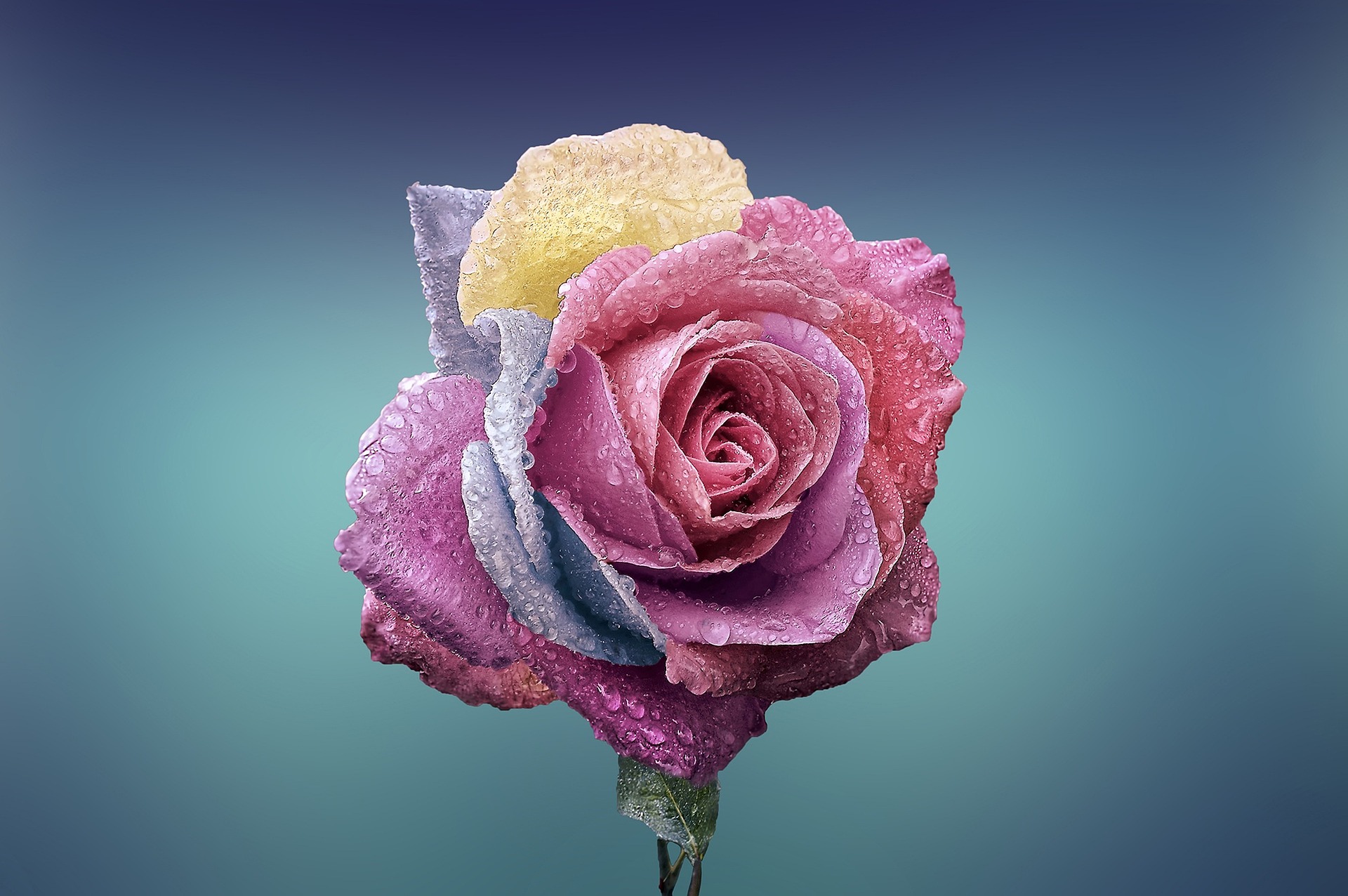
Why Your Roses Smell Nice
Hint—it’s mostly coincidence.
By Maxine Singer
Sign up for our monthly newsletter!
The appeal of many floral scents to humans is a fortunate byproduct: We were not even around when they appeared. And, for all the effort, commercial perfumes rarely smell like flowers. Expensive, fancy bottles labeled jasmine or gardenia may smell wonderful but they are sad substitutes for the real thing.
One reason is that flowers generally produce very large mixtures of different volatile molecules, as many as a thousand. Some of these fall into related chemical groups and although they differ very slightly in chemical structure, they can produce very different smells. In closely related flowers, the volatile molecules can vary both in relative amounts (reflecting differential regulation of the genes and gene products needed) and in their chemical structures (reflecting the activity of genes evolved to produce the enzymes needed for synthesis). It’s not easy to figure out which components of a mixture are important for attracting insects or birds or for achieving a perfume attractive to people. It is especially challenging because our own sense of smell depends on a complex set of nerve cells and often differs from one person to another. The manufacture of the odors depends on a plant’s genes, and the ability of animals, including ourselves, to smell those odors depends on the animals’ genes.
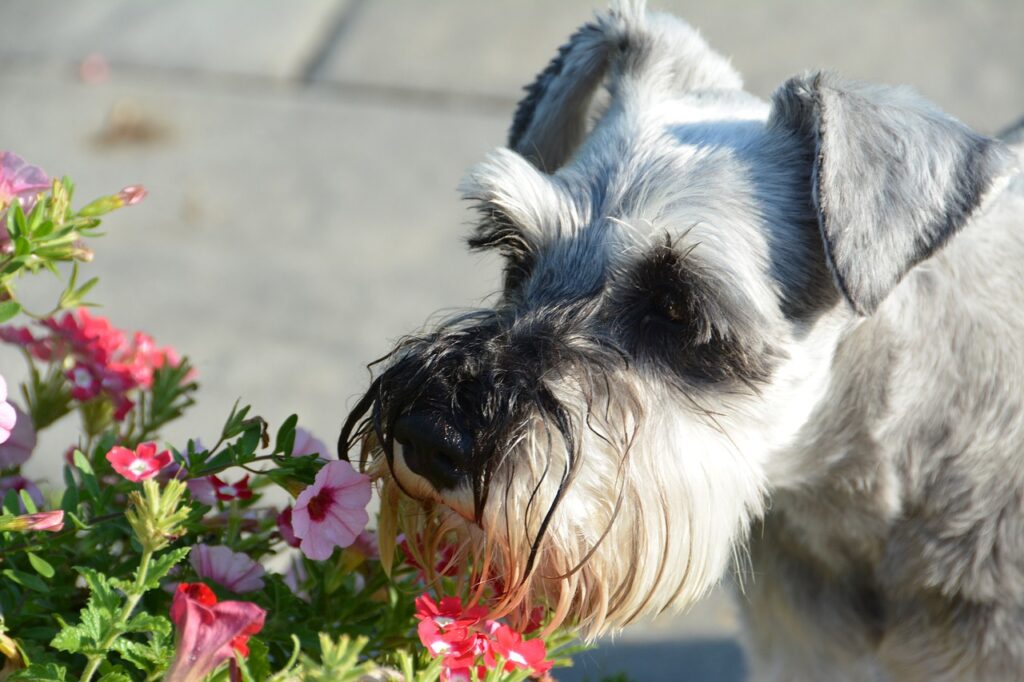
As with colors, the chemistry of volatile compounds that affect smell depends on the presence of genes that encode protein enzymes. These enzymes act in sequence to produce the complex scented molecules from precursor molecules whose presence depends on still other genes and enzymes. The relative amounts of the different molecules depend in turn on other genes that code for RNAs and proteins important for the regulation and modulation of the genes required to manufacture the scents.
When we smell a rose, we are picking up a mixture of several hundred different molecules. Each one of these is the result of a series of genes and the enzymes they code for that enable particular chemical reactions in the rose petals. Many of the volatile molecules are made from the amino acid phenylalanine.
Plants manufacture phenylalanine from simpler molecules, via a set of genes that code for the necessary protein enzymes. Phenylalanine is a close relative of tyrosine, the amino acid used by plants to manufacture the betalaine pigments, and it too is an “aromatic compound,” with a ring of carbon atoms. The difference in chemical structure between the two is simply that tyrosine has an additional oxygen (in the form of an –OH group attached to the carbon ring). In fact, mammals make tyrosine from phenylalanine (plants use another path). The list of pleasant-smelling molecules derived from phenylalanine and tyrosine is long.
Evolution has ensured that genes are most active when they are needed.
Plants make phenyalanine and tyrosine so that they can make proteins. But evolution, being opportunistic, makes use of the amino acids for other purposes too. Each use depends on evolving one or more additional genes that code for the enzymes that make the aromatics as well as the proteins and RNA needed to make sure that the genes are turned on in petals at the right time. A number of the aromatic volatiles will have originated from gene duplication events followed by mutation of the copies, a pattern we have now met several times. It is one of the most powerful ways in which variations become available on which natural selection can act.
To make a volatile aromatic from either of the amino acids phenylalanine or tyrosine requires chemical surgery on the amino acid by one or more reactions catalyzed by particular enzymes. One such reaction removes the amino group (–NH2) from the amino acid. If the starting molecule is phenylalanine, the result is a molecule called cinnamic acid; if the starting molecules is tyrosine, the result is coumaric acid. The only difference between cinnamic and coumaric acids is that coumaric acid has the same additional oxygen atom in the form of an –OH group as does tyrosine. Most, but not all, plant aromatics start out as one of these two molecules.
The name cinnamic acid shouldn’t be a mystery. It is what gives cinnamon its familiar smell. Cinnamon is the dried bark of certain evergreen trees of the genus Cinnamomum in the laurel family, which reminds us that many plant parts beside petals make aromatics. The enzyme that carries out the removal of the amino group from phenylalanine to produce the acid is called PAL, encoded by the gene PAL. Most plants have more than one PAL gene. The model plant Arabidopsis, for example, has four PAL genes, and these are active to different extents in different parts of the plant. It makes some sense to have several PAL genes because that same phenylalanine minus its amino group, as cinnamic acid, gives rise to many plant molecules beside volatiles. Among those molecules are lignin, the huge molecule found in tree wood, and the avonoid pigments used in coloring flowers. Some plants use PAL to start the long series of reactions leading to chalcone, the molecule that is eventually converted to the anthocyanin colors.
Another pathway to aromatics from phenylalanine involves two excisions on the amino acid. Here, both the amino (–NH2) and the acid (–COOH) groups which characterize it as an amino acid are removed. The resulting molecule is the starting point for making many other aromatic molecules. The level of the enzymes required to carry out this surgery in rose petals is most abundant in mature flowers, and in the late afternoon, when it is important to attract pollinating insects. Evolution has ensured that genes are most active when they are needed.
The identification of the gene responsible for the enzyme that removes the acid group from phenylalanine required real detective work. Plant genome data banks were searched for sequences that, by analogy with genes known in other organisms, might produce an enzyme that removes the acid group from phenylalanine. Scientists hit the jackpot when they found plant DNA sequences similar to the sequence of an animal gene that removes the acid group from a molecule called dopa that is related to phenylalanine. Familiar? That’s the same dopa that is used as a treatment for Parkinson’s disease. This DNA segment was most active in plants at the times and in the flower parts, petals, and ovaries when production of the volatile molecules from phenylalanine was highest. When activity of the gene in petunias was turned down experimentally in mutant plants, production of the aromatic stopped. The same was true for the rose version of the gene.
One aromatic molecule can contribute as much as 90 percent of the volatiles produced by the flowers.
The petunia and rose forms of this gene code for protein enzymes that are about 65 percent the same as animal enzymes that remove the acid portion from dopa and are similar to other plant enzymes that also remove the acid portion from other molecules. Together, all these genes belong to a family of related genes. It makes sense to conclude that they all evolved from some common ancestral gene.
Flowering plants have many more genes that code for enzymes required for production of other aromatics. Where did they all come from? Probably most, if not all of them, are related to genes that are important for other plant functions, and arose from past gene duplication events. That is what appears to have happened during the evolution of the genes responsible for the fragrant “tea” smell characteristic of popular tea roses. When ancient breeds of Chinese roses made their way to Europe late in the 18th century, it was recognized that they had a different perfume from European roses. Many years later, these unique smells were associated with particular compounds. By then, hybrids between Chinese and European roses had been bred. The hybrids, known as tea roses, are especially popular and one reason is their strong and appealing perfume, an inheritance from the Chinese parent of the hybrid. Among these perfumes one aromatic molecule (3,5-dimethoxytoluene, abbreviated to DMT) can contribute as much as 90 percent of the volatiles produced by the flowers. European rose petals don’t produce much, if any, of this molecule.
The DMT molecule is related to other plant aromatics constructed on a core ring of six carbon atoms, a few of which are decorated with assortments of carbon, hydrogen, and oxygen atoms. Various genes and enzymes give the plant the ability to make such decorated rings. Two enzymes coded for in Chinese rose genomes and active in Chinese rose petals can make the particular modifications leading to DMT. Why can’t the European roses do this? Because they don’t have the set of genes needed to make the proper modification. Two very closely related but distinct genes lead to the appropriate chemical changes in roses with a Chinese rose heritage somewhere in their past; they are called OOMT1 and OOMT2. Roses of purely European origins have only one of the two genes, while both proteins are needed to modify the aromatic ring in the right way to produce DMT. The 350 amino acids in the two enzymes OOMT1 and OOMT2 are 96 percent identical, and a change in just one amino acid out of the 350 is likely to be responsible for the difference in what they can do in the cells of petals. All this suggests that there was, initially, a single OOMT gene that became duplicated, and that one of the two copies then acquired mutations in its DNA and, as a consequence, changes to the amino acids of the protein enzyme for which it codes.
Which gene came first? If the OOMT genes in many different roses are compared, most have OOMT2 but only rose varieties with Chinese rose ancestors have OOMT1. The evolutionary tree of roses has features that make it likely that the Chinese roses appeared later in time than other roses. If so, that would be a strong clue that OOMT2 has been around for a longer time than OOMT1 and that it was OOMT2 that became duplicated.
Producing roses with smells that please people could not have been the reason for the success of this gene duplication and change by mutation. Why then did the new gene survive and succeed? It turns out to be bees: The important pollinators of roses seem to sense DMT.
Maxine Singer received her Ph.D. in 1957 from Yale University. She served on the editorial boards of the Proceedings of the National Academy of Sciences, and the Journal of Biological Chemistry and Science. She has received the Distinguished Presidential Rank Award, the National Medal of Science, and the Public Welfare Medal of the National Academy of Sciences.
Maxine Singer received her Ph.D. in 1957 from Yale University. She served on the editorial boards of the Proceedings of the National Academy of Sciences, and the Journal of Biological Chemistry and Science. She has received the Distinguished Presidential Rank Award, the National Medal of Science, and the Public Welfare Medal of the National Academy of Sciences.
From Blossoms: And the Genes That Make Them by Maxine F. Singer. Copyright © 2018 Maxine F. Singer. Published with permission from Oxford University Press. Previously excerpted in Nautilus.
Plantings
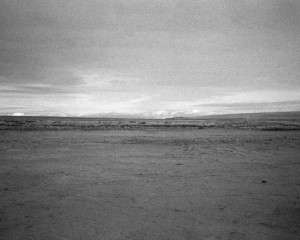
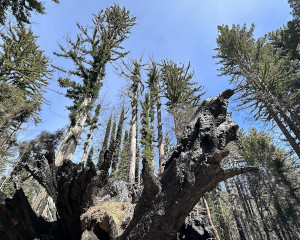
The Regenerating Power of Big Basin’s Redwoods
By Gayil Nalls
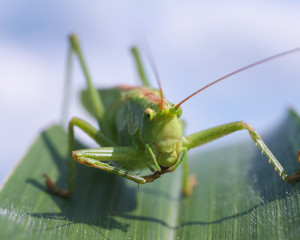
A warmer planet, less nutritious plants and … fewer grasshoppers?
By Amber Dance
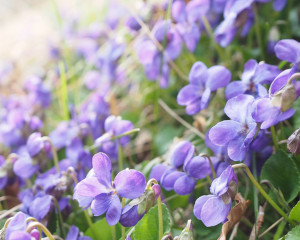
Viriditas: Musings on Magical Plants
By Margaux Crump
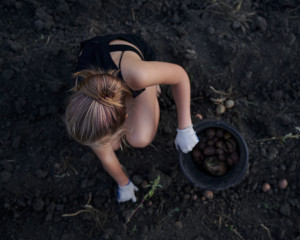
Seeing Ourselves in the Soil
By Jake Eshelman
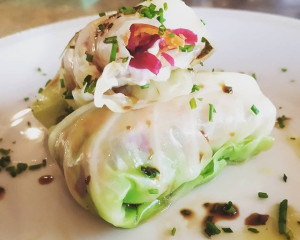
Eat More Plants Recipes:
Cabbage Roll with Cannellini Bean Hummus
By Sabina Cobbe
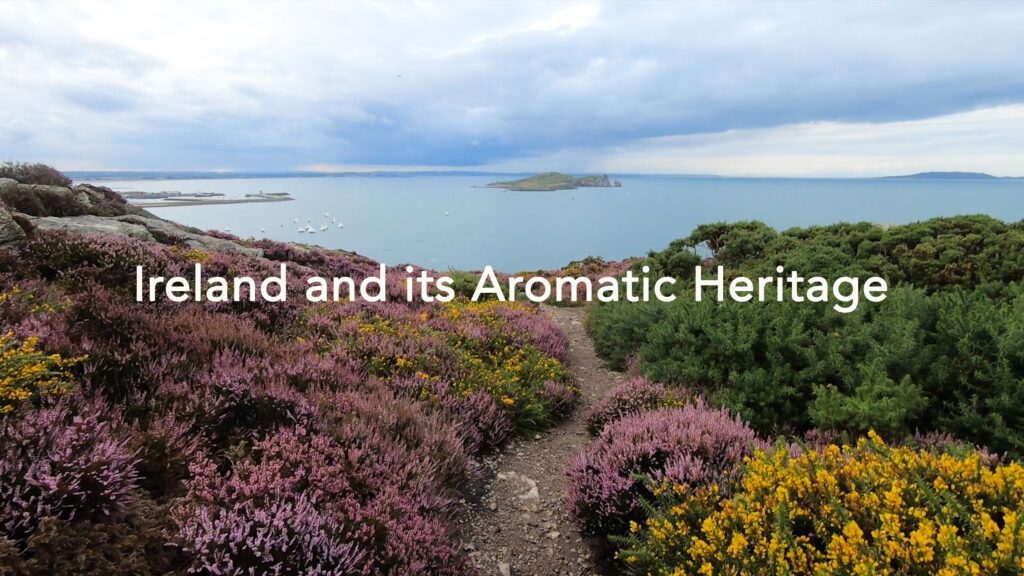
As Ireland transitions from the rich, smoky scent of peat-burning to a more sustainable future, its olfactory heritage is evolving. What will become the next iconic aromatic symbol of Ireland?
Click to watch the documentary trailer.