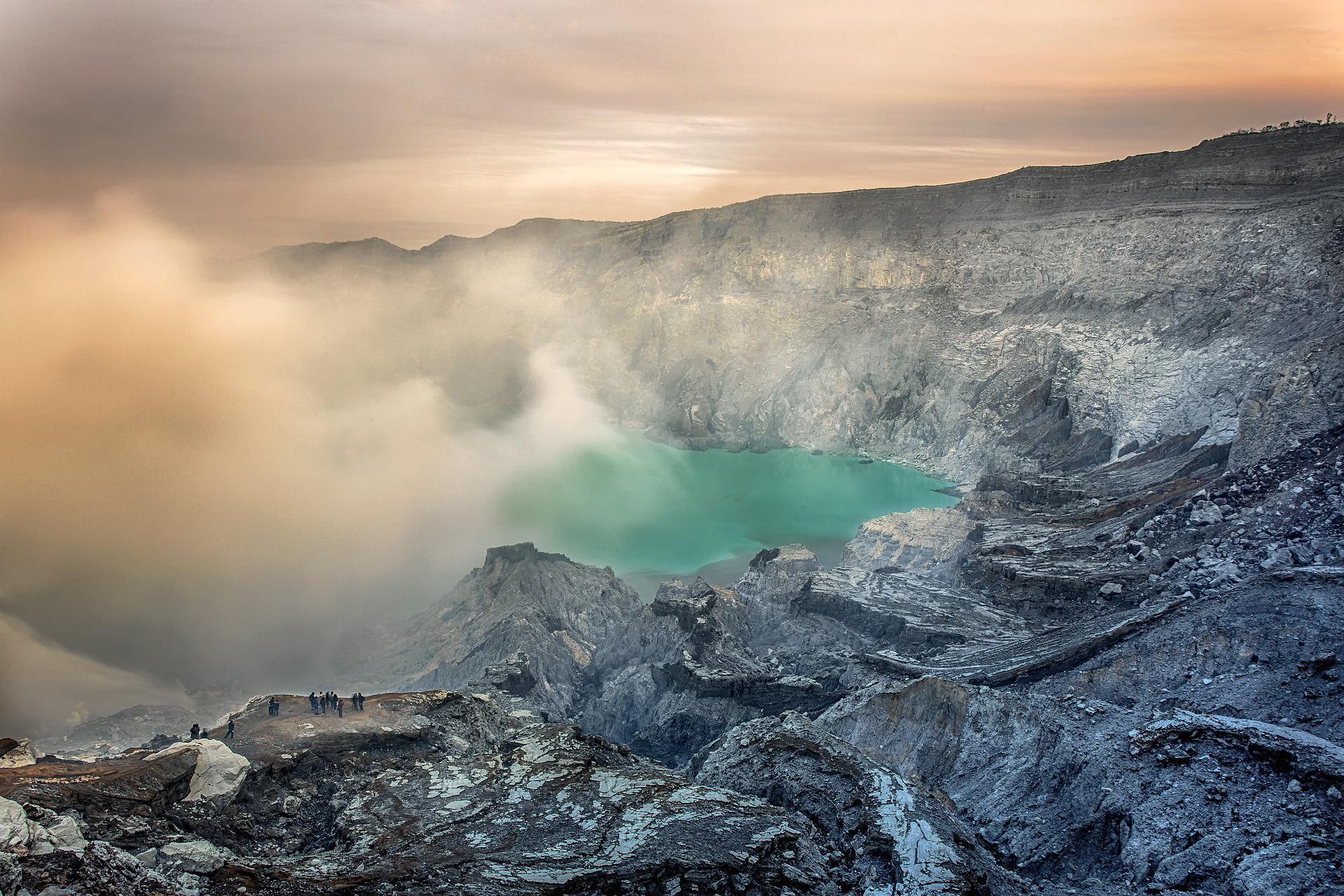
How We Perceive Nature Through Our Sense of Smell
By Andreas Keller
Sign up for our monthly newsletter!
This article offers a glance into the olfactory world of nature, in which we first discuss nature and its odors, then describe the scents that these odors produce. The difference between odors and smells is that odors are molecules in the air and smells are the mental representations of these odors in our minds. That there is an (often ignored) distinction between these two concepts can be a little confusing, because in color perception, we use the same word for both concepts. The term “color” refers to both the physical pigments in the environment and how they appear to us mentally. In comparison, the olfactory world is much more complex.
Part I: Nature and Its Odors
Most odors are generated by living things: plants, animals, and microorganisms. Some nonliving things also produce odors. Ozone and formaldehyde are formed by atmospheric processes, and hydrogen sulfide is produced by volcanoes, but essentially, the world was odorless before living things came along and started producing a wide variety of different odorous molecules. Roses alone release more than 250 different molecules. These molecules contribute in complex ways to the rose smell. Many of them contribute very little, while the mixture of beta-damascone, beta-damascenone, and (-)-cis-rose oxide, which combined make up less than 1% of the total volume of rose essential oil, is necessary for the rose’s characteristic scent.
The complexity of the possible mixtures of odor molecules is staggering. There are over 30 billion odorous molecules and around 2 million of them have so far been found in nature.
A rose does not have an odor, it makes an odor.
A common misconception, dating back to Aristotle’s writing on scent, is that odor molecules are components of their source objects and detach to become airborne, like water molecules evaporating from a lake. In reality, rose odor molecules are actively produced in certain parts of the plant, transported to other structures where they are stored, and then released in appropriate situations. Enzymes that have evolved specifically for this activity make it feasible for this energy-intensive process to occur. A rose does not have an odor, it makes an odor. And it does make that odor for a reason. It performs an adaptive function for the rose rather than being a byproduct of being a rose.
Odors as Signals
A well-known function of flower odors is to attract pollinators. The odor is a signal the flowers send to potential pollinators, usually insects. But pollinators are not the only recipients of plants’ odor signals. Some plants also use odors to signal to other plants. Black Walnut (Juglans nigra) gives off a molecule called juglone that repels other plants, thereby reducing competition for space, water and nutrients. Other trees, such as sugar maples, sycamore, and red oak also produce allelochemicals to assure their survival. When grass gets damaged, it releases molecules that elicit the typical “cut-grass” smell. These molecules are signals for other grass that the plant is under attack. In animals, it would be called an alarm pheromone.
Orchids are masters of mimicking smells found in the natural world and using them for the purpose of getting pollinated.
Potential predators are also common recipients for plants’ odor signals. For example, caraway seeds make the chemical carvone that gives them their distinct scent in order to repel insects and other potential animals which may eat them. Ironically, humans like the flavor of caraway and producing carvone has increased caraway’s danger of being eaten by people. The same dynamic is known for the molecules that give chili peppers their spiciness. Their function is to deter animals from eating them, but humans have developed a masochistic liking for the taste and started eating chili peppers.
When a plant or animal dies, bacteria break down the large, synthesized odor molecules into smaller ones. These smaller molecules are responsible for the smell of decay or fermentation. They do not have a function but are a byproduct of the bacteria breaking down larger molecules. Plants may then use the small molecules produced by bacteria again to make larger molecules and complete the cycle.
Citrus Fruits
Different plants create various odor molecules, and as a result, they all have a distinct smell. However, sometimes plants have similar smells because they produce similar mixtures of molecules. The fruits of citrus plants are a well-understood case study for the contribution of odors to smells. All the citrus fruits available in a supermarket—oranges, limes, lemons, grapefruits—are the result of humans crossing the four naturally-occurring citrus fruits: pomelos, true mandarins, citrons, and small-flowered papedas. For generations, farmers have hybridized these species to generate hundreds of new variants that combine the characteristics of the four ancestral citrus fruits. The cross between a pomelo and a mandarin, for example, results in a sweet orange, and if a sweet orange is crossed again with a pomelo, the result is a grapefruit. In this area defined by the ancestral species, fruits may be moved within this spectrum. These ancestral species produce different odors because they evolved different odor-producing enzymes. As the plants are hybridized, these enzymes can be combined in novel ways, thereby changing what odor molecules are being produced. The scents of various citrus fruit varieties differ as a result of the recombination of odor synthesis pathways. All citrus fruits share many of the odorous molecules that they release. For example, limonene, a molecule with a citrusy, fruity smell, makes up more than 50% of the essential oils of most citrus fruits.
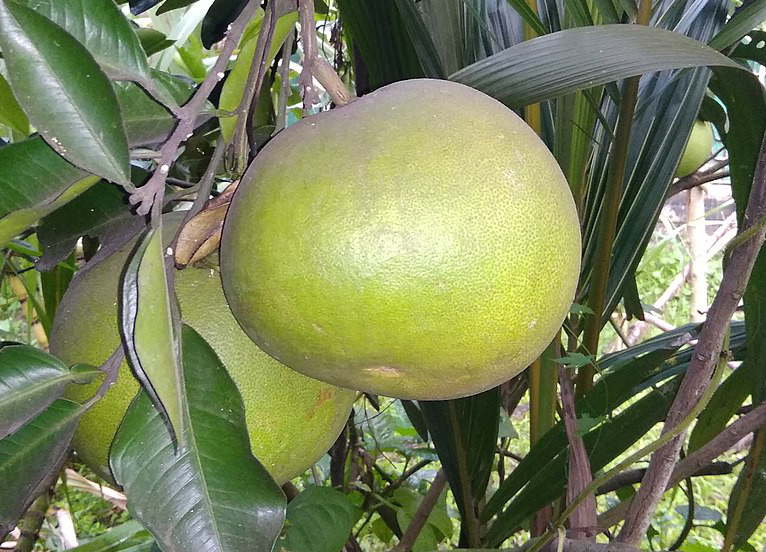
Common elements like limonene are responsible for the olfactory qualities that are shared by all varieties of citrus fruits. But of course, not all citrus fruits have the same smell, it is generally easy to distinguish the smell of an orange from the smell of a grapefruit. These differences in the odor of citrus fruit varieties are due to molecules in the mixture that are only found (at relevant concentrations) in those specific kinds. The molecule nootkatone, for example, is only found in grapefruit, and it is responsible for the characteristic bitter aroma of grapefruits. The combination of shared and exclusive odor molecules explains the similar yet unique smells of citrus fruits.
Orchids
All citrus fruits are closely related, but there are also cases of widely different plants which produce the same odors. These are examples of convergent evolution, which happens when unrelated species independently evolve the same solution to a problem. The textbook example is the independent evolution of wings for flying in bats, birds, and insects. When it comes to plants, orchids are well known for producing a wide variety of odors that often mimic those of other plants. There are orchid species that smell like piña colada, white chocolate, vanilla, strawberry, or rhubarb. These orchid species have evolved ways of synthesizing the same molecules, and hence comparable scents of other unrelated plant species.
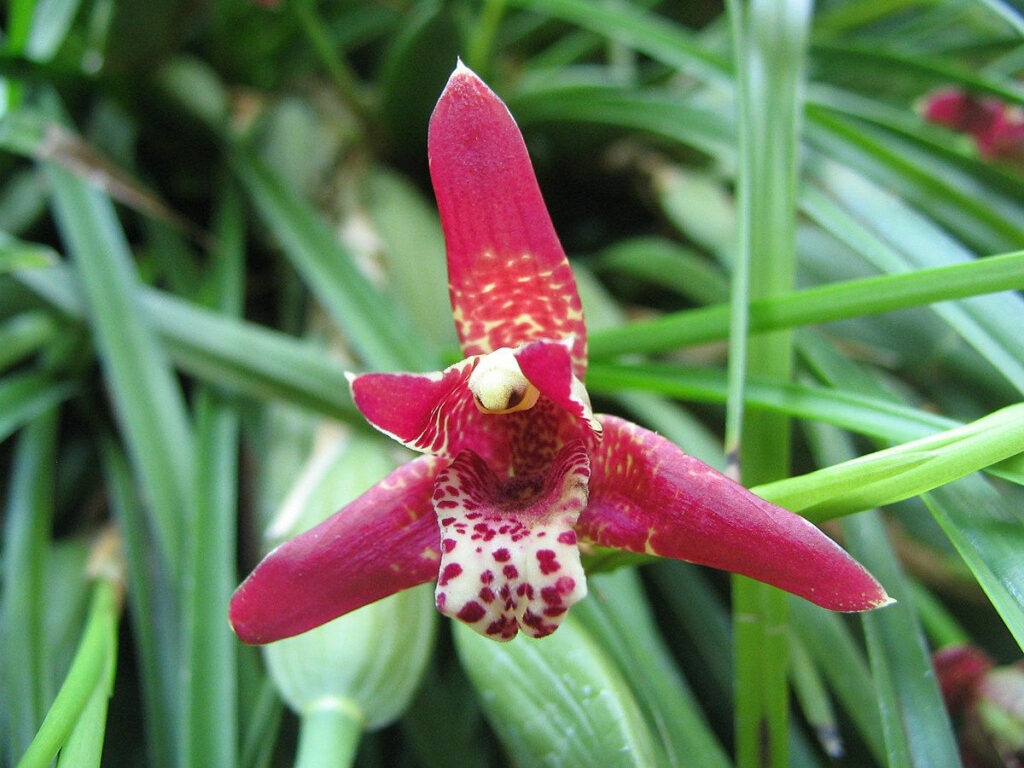
Not all orchids mimic the smell of other plants. Some orchids smell like rotting flesh to attract pollinating insects that usually eat decaying meat. Others attract bugs by feigning to be a female who is available and emitting an odor similar to the pheromones of insects. Orchids are masters of mimicking smells found in the natural world and using them for the purpose of getting pollinated. There is a type of orchid in Korea that mimics the alarm pheromone of bees, which attracts insects that prey on bees. The predatory insects smell what they think is an injured bee and, searching for the easy prey, get trapped in the orchid and pollinate it.
Part II: Smelling Nature
For an odor to elicit a smell, it has to be smelled by somebody. This happens when the odor molecules emitted by plants reach our nose. On the very top of the nostrils, between the eyes, the olfactory nerve enters the nasal cavity where it terminates in the olfactory epithelium. The olfactory nerve consists of different types of sensory neurons. Each type expresses one of hundreds of different odorant receptors, which are the molecules that bind to odor molecules. The neurons of the olfactory nerve, which are directly exposed to the unfavorable environment of the nasal cavity, continuously die and regrow.
For an odor to elicit a smell, it has to be smelled by somebody. This happens when the odor molecules emitted by plants reach our nose.
Combinatorial Code
In our nasal cavities and the air surrounding us, various combinations of odorous airborne molecules are present at any one time. Different molecules from this mixture bind to various odorant receptors, thereby activating the sensory neuron. The sensory neuron then sends a signal up the olfactory nerve, through the skull, into a brain structure called the olfactory bulb. The olfactory bulb consists of spherical structures called glomeruli. In each glomerulus, sensory neurons of the same type converge. Every molecule will activate a combination of receptors. To give a hypothetical example, vanillin may activate receptors 73, 115 and 299, the activation of the receptor combination 73, 115, 299 therefore signals to the brain that vanillin molecules are being encountered. This is known as the “combinatorial code” of olfaction: each type of odor molecule activates several receptors, and each receptor is activated by several types of odor molecules, resulting in a unique combination of activated receptors for each type of odor molecule.
The artist Sissel Tolaas once isolated bacteria from David Beckham’s dirty gym socks and used them to make cheese.
Odorant Receptors
The odorant receptor gene family is the largest in our genome. Humans have, depending on the counting method, 400 or 800 odorant receptor genes, compared to only three-color pigments. Whether we count 400 or 800 odorant receptor genes depends on whether pseudogenes are counted. Pseudogenes are genes that have accumulated mutations that rendered them non-functional. When scientists first sequenced the human genome and realized that half of our odorant receptor genes are pseudogenes, they speculated that this was a consequence of the declined importance of olfaction for our species. If our sense of smell no longer plays a role in our survival and reproductive fitness, there is no selective pressure against deleterious mutations in odorant receptor genes and these mutations can accumulate, turning the genes into pseudogenes. However, after the genomes of various other animal species had been sequenced, it became clear that odorant receptor pseudogenes are very common in almost all species, including those well-known to rely heavily on their sense of smell.
Specific Anosmias
Many odorant receptor genes are found as a functional variant in some people and as a non-functional variant in others. In these cases, some of us carry the gene while others carry the pseudogene. People who carry the non-functional variant sometimes have reduced sensitivity to the odor molecules the receptor binds. This is a condition known as “specific anosmia” or “partial smell blindness.” Somebody with specific anosmia has a normal sense of smell except that they cannot smell a specific group of molecules or smells, such as musk. Specific anosmia is the olfactory version of partial color blindness (such as red-green color blindness), which is also caused by genetic variation in the gene for the protein interacting with the stimulus. However, there is a conceptually very important difference between specific anosmia and color blindness: in color perception, over 90% of the population have functionally the same set of photoreceptors. In olfaction, everyone has a unique collection of odor receptors, with the exception of identical twins. There is no “normal” set of receptors and therefore also no standard way of perceiving odors. As a result, an odor does not have a smell. Instead, a smell is assigned to an odor, and which smell somebody assigns to a specific odor depends on which set of receptors they are smelling it with. If two people assign different smells to the same odor, there is no principled way of determining who is right. Because they can both be right.
We don’t normally recognize that we have a specific anosmia because almost all odors we encounter are mixtures and when we are less sensitive to a component of the mixture, we are still able to smell it. Differences in the perception of the aroma of cilantro, and inability to smell the characteristic odor of urine after eating asparagus, are two examples of a specific anosmia that has noticeable effects on perception and behavior in everyday life. Another example is the specific anosmia to the odorous steroid androstenone and related components. These components, which are odorless for many, but have an unpleasant smell of sweat and urine for most, are found in the meat of uncastrated male pigs. People with this specific anosmia are less likely to detect a taint in such meat and are therefore more likely to eat it.
Influence of Background Beliefs
How we perceive a given odor through our sense of smell depends strongly on our odorant receptor variants. How we judge odors on the other hand is deeply affected by the context and our previous experiences. The various reactions people have to certain odors provide insight into how deeply these aspects might influence opinions—like pork, fermented food, and spices like garlic—or cigarette smoke in different populations.
The influence on background beliefs on olfactory judgments has been confirmed in controlled studies. In one of those studies, subjects were asked to rate the smell of isovaleric acid, a molecule that is found both in the odor of parmesan cheese and dirty gym socks because it is produced by bacteria that thrive in both environments. (The artist Sissel Tolaas once isolated bacteria from David Beckham’s dirty gym socks and used them to make cheese.) For the study, one group of the subjects who were judging the smell of isovaleric acid were under the impression that the source of the molecule was sweat, while another group was under the impression that the source was cheese. Depending on what they believed to be the source of the odor (the real source was a chemical factory in which the isovaleric acid was synthesized), subjects assigned dramatically different pleasantness ratings to the odor.
Influence of Other Modalities
In another study that demonstrates the tremendous impact of non-olfactory factors on peoples’ judgments of odors, researchers used students of wine making at a school in Bordeaux as their subjects. These wine experts were asked to smell (but not taste) three different wines and describe their aroma by picking descriptors from a list. One wine was a red wine, the other a white wine, and the third the same white wine but colored red with odorless food coloring. The subjects’ description of the odor of the white wine with the red coloring was much more similar to the description of the odor of the red wine than to the description of the odor of the white wine. This means that a wine’s description of its aroma was influenced more by its color than by its smell.
While the results of these experiments are clear and dramatic, the interpretation of the results is difficult. Does the white wine actually smell different when it is colored red, or does it smell the same but the smell is judged differently? I believe the latter is the case. The students knew which descriptors are commonly used for red wines: tar, leather, plum, cherry—and which descriptors are commonly used for white wines: honey, apricot, apple, hay. They did not want to look foolish by using white wine descriptors to describe a red wine. Their background knowledge, rather than helping them with the task, was entrenched enough to overrule the sensory evidence. Designing an experiment that would confirm or refute this interpretation of the result is challenging, though.
Influence of Previous Experiences
The studies with the isovaleric acid with various labels and the wine with different colors show the impact of background beliefs and sensory input from other modalities on accounts of olfactory perception. In addition, these reports are shaped by previous experiences with the smell. The pleasantness of a smell associated with a food or drink that has caused food poisoning, for example, will be judged very differently before and after it caused the discomfort. These effects can be astonishingly long-lasting and difficult to overcome. Even scent experiences in the womb, before being born, impact lifelong attitudes towards the experienced odors. Researchers in France demonstrated this by recruiting pregnant women and dividing them into two groups. One group was instructed to regularly eat anise-flavored candy throughout their pregnancy, whereas the other group was instructed to avoid such candy. When the newborns, minutes after they were born, were tested for their response to anise odor, they demonstrated the predicted differences between the two groups. Babies of women who consumed anise-flavored candy during pregnancy displayed attraction and curiosity towards the odor whereas babies in the other group were indifferent to the scent.
This short glimpse into the world of odors and smells shows that the odors in nature, the mental representations elicited by them, and the verbal reports about the odors are more complex and multilayered than we usually appreciate. The smells of nature, especially those that are in danger of disappearing, are deserving of our respect and appreciation and we must ensure that they can be experienced by future generations.
Dr. Andreas Keller received his PhD in Genetics from the Julius-Maximilian-University in Wuerzburg, Germany, and holds a second PhD in Philosophy from the City University of New York. He continues research in olfaction at Rockefeller University and is the founder and owner of the NYC art gallery Olfactory Art Keller.
Special Holiday Offer!
This holiday season, give the gift of inspiration and action.
For a limited time, purchase the set of three annual issues at a special flat rate:
- $64 (including shipping) within the US
- $89 (including shipping) outside the US
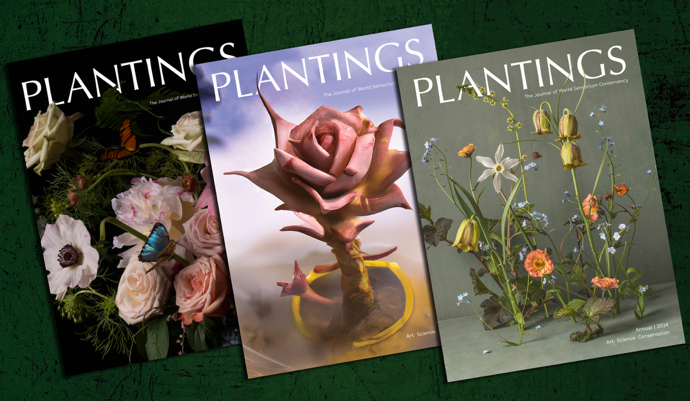
Enjoy three years of transformative content that makes a difference. Don’t miss this opportunity to share meaningful stories and ideas that inspire change!
Offer valid till January 15.